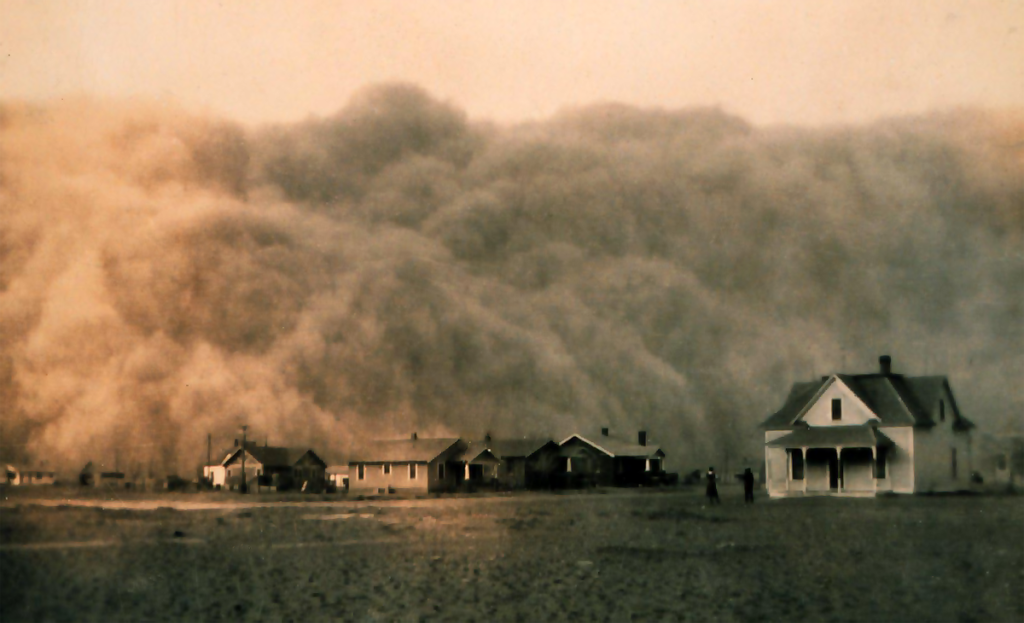
A new study using a reconstruction of North American drought history over the last
1,000 years found that the drought of 1934 was the driest and most widespread of the
last millennium.
Using a tree-ring-based drought record from the years 1000 to 2005 and modern records, scientists from NASA and Lamont-Doherty Earth Observatory found the 1934 drought was 30 percent more severe than the runner-up drought (in 1580) and extended across 71.6 % of western North America. For comparison, the average extent of the 2012 drought was 59.7 percent.

This photo shows a farmer and his two sons during a dust storm in Cimarron County, Oklahoma, 1936.
The 1930s Dust Bowl drought had four drought events with no time to recover in between: 1930-31, 1934, 1936 and 1939-40. Image Credit: Arthur Rothstein, Farm Security Administration
“It was the worst by a large margin, falling pretty far outside the normal range of variability that we see in the record,” said climate scientist Ben Cook at NASA’s Goddard Institute for Space Studies in New York. Cook is lead author of the study, which will publish in the Oct. 17 edition of Geophysical Research Letters.
Two sets of conditions led to the severity and extent of the 1934 drought. First, a high-pressure system in winter sat over the west coast of the United States and turned away
wet weather – a pattern similar to that which occurred in the winter of 2013-14.
Second, the spring of 1934 saw dust storms, caused by poor land management practices, suppress rainfall.
Five new NASA Earth science missions are launching in 2014 to expand our understanding of Earth’s changing climate and environment. NASA’s “Earth Right Now” website
“In combination then, these two different phenomena managed to bring almost the
entire nation into a drought at that time,” said co-author Richard Seager, professor at the Lamont-Doherty Earth Observatory of Columbia University in New York. “The fact that it was the worst of the millennium was probably in part because of the human role.”

Brown colors of the Palmer Drought Severity Index, or PDSI, indicate strong drought conditions across the United States in the summer of 1934. PDSI was calculated from monthly averages of precipitation, temperature and other factors from 1934, available from the Climate Research Unit.
Image Credit: GISS/Lamont-Doherty
According to the recent Fifth Assessment Report of the Intergovernmental Panel on Climate Change, or IPCC, climate change is likely to make droughts in North America worse, and the southwest in particular is expected to become significantly drier as are summers in the central plains. Looking back one thousand years in time is one way
to get a handle on the natural variability of droughts so that scientists can tease out anthropogenic effects – such as the dust storms of 1934.
“We want to understand droughts of the past to understand to what extent climate
change might make it more or less likely that those events occur in the future,” Cook said.
The abnormal high-pressure system is one lesson from the past that informs scientists’ understanding of the current severe drought in California and the western United States.
“What you saw during this last winter and during 1934, because of this high pressure in the atmosphere, is that all the wintertime storms that would normally come into places like California instead got steered much, much farther north,” Cook said. “It’s these wintertime storms that provide most of the moisture in California. So, without getting
that rainfall it led to a pretty severe drought.”
This type of high-pressure system is part of normal variation in the atmosphere, and whether or not it will appear in a given year is difficult to predict in computer models of the climate. Models are more attuned to droughts caused by La Niña’s colder sea surface temperatures in the Pacific Ocean, which likely triggered the multi-year Dust Bowl drought throughout the 1930s. In a normal La Niña year, the Pacific Northwest receives more rain than usual, and the southwestern states typically dry out.
But a comparison of weather data to models looking at La Niña effects showed that the rain-blocking high-pressure system in the winter of 1933-34 overrode the effects of La Niña for the western states. This dried out areas from northern California to the Rockies that otherwise might have been wetter.
As winter ended, the high-pressure system shifted eastward, interfering with spring and summer rains that typically fall on the central plains. The dry conditions were exacerbated and spread even farther east by dust storms.
“We found that a lot of the drying that occurred in the springtime occurred downwind from where the dust storms originated,” Cook said, “suggesting that it’s actually the dust in the atmosphere that’s driving at least some of the drying in the spring and really allowing this drought event to spread upwards into the central plains.”

A “black blizzard” dust storm in South Dakota, 1934.
Image Credit: National Archives FDR Library Public Domain Photographs
Dust clouds reflect sunlight and block solar energy from reaching the surface.
That prevents evaporation that would otherwise help form rain clouds, meaning
that the presence of the dust clouds themselves leads to less rain, Cook said.
“Previous work and this work offer some evidence that you need this dust feedback
to explain the real anomalous nature of the Dust Bowl drought in 1934,” Cook said.
Dust storms like the ones in the 1930s aren’t a problem in North America today.
The agricultural practices that gave rise to the Dust Bowl were replaced by those that minimize erosion.
Still, agricultural producers need to pay attention to the changing climate and adapt accordingly, not forgetting the lessons of the past, said Seager. “The risk of severe mid-continental droughts is expected to go up over time, not down,” he said.
›The worst North American drought year of the last millennium: 1934
Benjamin I. Cook, Richard Seager, Jason E. Smerdon
First published: 23 September 2014
https://doi.org/10.1002/2014GL061661
Abstract
During the summer of 1934, over 70% of western North America experienced extreme drought, placing this summer far outside the normal range of drought variability and making 1934 the single worst drought year of the last millennium. Strong atmospheric ridging along the West Coast suppressed cold season precipitation across the Northwest, Southwest, and California, a circulation pattern similar to the winters of 1976–1977 and 2013–2014. In the spring and summer, the drying spread to the Midwest and Central Plains, driven by severe precipitation deficits downwind from regions of major dust storm activity, consistent with previous work linking drying during the Dust Bowl to anthropogenic dust aerosol forcing. Despite a moderate La Niña, contributions from sea surface temperature forcing were small, suggesting that the anomalous 1934 drought was primarily a consequence of atmospheric variability, possibly amplified by dust forcing that intensified and spread the drought across nearly all of western North America.
Key Points
The 1934 drought was the most widespread and severe event of the last millennium.
Atmospheric variability caused winter drying; dust intensified drying in spring.
Despite a strong La Niña, SST forcing played only a small role.
1 Introduction
The Dust Bowl drought of the 1930s was one of the worst environmental disasters
in United States history, causing widespread crop failures, land degradation, human migration, and a permanent transformation in the social, economic, and agricultural structure of the Great Plains [Hansen and Libecap, 2004; Worster, 1979; Seager et al., 2008].
The inception of this event is linked to sea surface temperature (SST) variations in the tropical Pacific [Schubert et al., 2004a, 2004b; Seager et al., 2008], while the intensity and atypical spatial pattern of the drought is attributed to random atmospheric variability [Hoerling et al., 2009] and/or dust aerosol and land surface feedbacks:
[Cook et al., 2008, 2009; Schubert et al., 2004a]. Even by the standards set by the multiple years of the Dust Bowl drought, however, the summer of 1934 stands out as exceptionally severe.
While the term “Dust Bowl” was not coined until the following year, 1934 directly set the stage for 1935 and the subsequent years that would be characterized by extensive drought, widespread dust storms (including the Black Sunday storm of 14 April 1935), and the formation of the Soil Conservation Service [Worster, 1979]. The drying that fed the 1934 drought began the previous fall [Murphy, 1935], with major precipitation deficits in California, the Northwest, the Southwest, and across the Southern Plains.
By the spring of 1934, the drying shifted to the Central Plains and Midwest.
Importantly, these precipitation deficits occurred during the primary seasons of moisture supply for the various regions (i.e., winter and early spring in California and the West and spring over the Central Plains and Midwest), ensuring little likelihood of relief with the onset of summer. Accompanying the precipitation anomalies were five major dust storms originating in the Central Plains from November 1933 to May 1934 [Mattice, 1935], caused in part by anthropogenic land use practices [Hansen and Libecap, 2004; McLeman et al., 2014].
Three of these events occurred in April 1934 from 9–12, 18–20, and 21–24, which collectively spread dust as far east as North Carolina and Florida, while the largest dust storm on 9–12 May spread dust across most of the United States east of the Central Plains [Mattice, 1935]. By the end of 1934, it was estimated also that 65% of the total area of the Great Plains had been damaged by wind erosion, with 15% severely affected [Hansen and Libecap, 2004].
Beyond the dust storms and wind erosion, the drought had severe impacts on agriculture and water resources, as documented in a report issued by the federal government the following year [Murphy, 1935]. Through the summer of 1934, conditions for pasture, corn, and tame hay (hay cut from cultivated grasses) were considered extremely poor over most of western North America (WNA), from Texas and New Mexico and up through Montana and the Dakotas. Reservoir levels were low across WNA, averaging ∼55% of capacity in Nevada, ∼40% in California, ∼22% in New Mexico, ∼18% in Idaho, and ∼13% in Colorado and Wyoming.
By the end of the summer of 1934, over 1100 counties had received an emergency drought designation. Ultimately, the effects of the drought were so severe that in June 1934, President Roosevelt requested <DOLLAR/>525 million in drought relief from Congress, over half (<DOLLAR/>275 million) of which was intended to relieve the livestock industry by means of providing emergency feed, buying starving animals from farmers, and slaughtering excess herds for food relief [Worster, 1979].
Previous studies have analyzed SST and land surface forcing during the persistent, multiyear Dust Bowl drought [Cook et al., 2008, 2009; Hoerling et al., 2009;
Schubert et al., 2004a, 2004b; Seager et al., 2008], but no study to date has conducted a comprehensive analysis specifically for the exceptional drought year of 1934.
Moreover, while regional drought variability in North America is understood reasonably well [e.g., Schubert et al., 2008; 2009; Seager et al., 2005a; Seager and Hoerling, 2014], we know much less about the dynamics underlying multiregion, pancontinental drought events [Cook et al., 2014] like 1934; events that, because of their extent, often have much larger social impacts [e.g., Hoerling et al., 2014]. Here we analyze the summer drought of 1934 by investigating the seasonal evolution of the underlying climate anomalies, placing this drought within the context of the paleoclimate record, and drawing lessons regarding what might be learned to better understand the severity and occurrence of current and future pancontinental droughts.
2 Materials and Methods
Our analysis is focused on the climate anomalies (temperature and precipitation) and dynamics during the “water year” (October 1933 to September 1934) leading up to and during the summer drought of 1934. The main seasons of moisture supply are different across the various regions of WNA: for example, most precipitation falls in winter in the Southwest and along the West Coast, while spring and summer dominate the Central Plains. It is therefore likely that the drought in each region of WNA will have a different meteorological and dynamical explanation, and thus, a consideration of the climate anomalies across the entire water year is required.
An updated version of the North American Drought Atlas (NADA) is used to characterize the 1934 drought and place this event within the context of North American drought variability over the last millennium [Cook et al., 2004, 2010]. The NADA is a tree ring-based gridded reconstruction (0.5° lat/lon) of summer season (June-July-August; JJA) Thornthwaite-Based Palmer Drought Severity Index (PDSI). PDSI is a normalized index
of drought, integrating changes in moisture supply (precipitation) and demand (evapotranspiration) with a memory timescale of about 12–18 months (meaning that summer season PDSI will depend, at least partially, on climate anomalies in prior months and seasons). Negative PDSI values indicate drier than average conditions; positive values indicate wetter than average conditions. In the NADA, all anomalies are relative to the average baseline for 1931–1990, and we use data from the NADA spanning 1000–2005 Common Era (C.E.).
We calculate temperature and precipitation anomalies from the latest version (3.21) of
the climate grids provided by the Climate Research Unit (CRU) at the University of East Anglia [Harris et al., 2014]. The CRU climate grids are monthly average fields of climate variables that are interpolated from station observations to a uniform 0.5° lat/lon grid, with temporal coverage spanning the entire twentieth century (1901–2012). SST anomalies are taken from the HadISST data set [Rayner et al., 2003] (1870 to present). We use 500 hPa geopotential heights from version 2 of the Twentieth Century Reanalysis (1871–2012) to investigate the dynamics [Compo et al., 2011]. For a comparison with the winter of 2013–2014, we also use dynamical fields from the National Centers for Environmental Prediction-National Center for Atmospheric Research (NCEP-NCAR) reanalysis [Kalnay et al., 1996] (1948 to present).
To make things consistent with the base period in the NADA, all anomalies are calculated relative to the mean climatology for 1931–1990, except for the NCEP-NCAR reanalysis, which uses a baseline of 1948–1990.
3 Results
3.1 The Drought
Drought during the summer of 1934, as reflected in the PDSI anomalies, was intense and widespread across much of North America (Figure 1), extending from the West Coast to the Great Lakes and from Texas to Montana. The tree ring based PDSI estimates in the NADA (Figure 1, left) are largely corroborated by PDSI values independently calculated from the CRU climate grids (Figure 1, right).
Area-averaged NADA PDSI for western North America (WNA: 125°W–85°W, 30°N–49°N) in 1934 was −4.11, the single most intense drought year of the last millennium (Figure 2, top). This magnitude of drought in WNA was a departure of over 3.5 standard deviations from the long-term mean (1000–2005 C.E.) and exceeded the magnitude of the second most intense drought year in WNA (1580, PDSI = −3.19) by almost 30%.

Figure 1 Open in figure viewer PowerPoint
Summer season (JJA) Palmer Drought Severity Index (PDSI) for 1934 from (left) the North American Drought Atlas and calculated from (right) the CRU 3.21 climate grids.

Figure 2 Open in figure viewer PowerPoint
For western North America (125°W–85°W, 30°–49°), (top) area-averaged PDSI and (bottom) percent area with PDSI anomalies less than or equal to −3, indicating extreme drought.
In addition to being exceptionally intense, 1934 was also an outlier in terms of drought extent, with extreme drought (PDSI ≤−3) covering 71.6% of WNA, the most extensive summer of extreme drought by a broad margin (Figure 2, bottom). By comparison, the second most extensive extreme drought year was 1956, when 49.3% of WNA was affected. The NADA ends in 2005 and therefore cannot be used to compare 1934 to more recent extreme drought years, such as 2011 and 2012. The National Climatic Data Center (NCDC) nevertheless tracks the percent area of the United States in “moderately to extremely dry” conditions over the instrumental period (1895 to present; see Acknowledgements for data URL).
According to this metric, drought extent was above 70% for May through September 1934, averaging 77.2% in JJA. By comparison, the average for JJA of 2012 was only 59.7%, with a maximum extent in July of 61.8%. The average extent in JJA of 2011 was even lower at only 28.9%, indicating that 2011, while extreme, was highly localized (predominantly in the Southwest and Southern Plains) [Seager et al., 2014]. These results confirm the NADA analysis, further supporting our observation that 1934 was the worst single year of drought in WNA of the last 1000 years.
3.2 La Niña Forcing
Cold SST anomalies in the eastern tropical Pacific developed in the fall of 1933
(Figure S1), a transition to La Niña conditions typically associated with suppressed precipitation across the Southwest and Southern Plains [Ropelewski and Halpert, 1987; Seager et al., 2005b, 2014]. During October–December (OND) the NINO 3.4 index anomaly was −0.98 K, weakening slightly to −0.85K during January–March (JFM) in 1934. Despite the moderately strong
La Niña, circulation over North America during OND (Figure S2) and JFM (Figure S3) diverged substantially from the canonical La Niña response.
During a typical La Niña, a broad, zonally oriented band of high pressure extends across the Southwest and Southern Plains [Seager et al., 2010, 2014; Trenberth et al., 1998]. Circulation during the fall and winter of 1933–1934, however, was characterized by an upper-level ridge centered over the West Coast and an accompanying trough over the Northeast (Figure 3). This suggests that despite its strength, the 1933–1934 La Niña event was a relatively minor contributor to the precipitation deficits preceding the 1934 drought, even though persistent La Niña forcing was likely important to establishing the Dust Bowl as a long-term, multiyear event [e.g., Schubert et al., 2004a; Seager et al., 2008].

Figure 3 Open in figure viewer PowerPoint
(Left column) Precipitation (mm d−1), (middle column) temperature (K), &
(right column) 500 hPa height (m) anomalies during the fall and winter of 1933–1934.
3.3 Winter and Early Spring
Cold season precipitation was reduced across much of WNA during the winter of 1933–1934 (Figure 3, left column), with major deficits in California, the Northwest, and the eastern half of the United States. Along with the drying, warm temperatures prevailed across most of WNA, while most of eastern North America was anomalously cold (Figure 3, middle column).
Such a dipole configuration, characterized by a ridge over the Gulf of Alaska or along the West Coast and a trough over northeast North America, would have acted to suppress precipitation over much of WNA and favored the advection of cold air from high latitudes into the eastern part of the continent.
These circulation and climate anomalies strongly resemble the most recent winter of 2013–2014 (Figure S4) when a similar, but more persistent, circulation pattern intensified the ongoing drought in California and the Southwest and caused extreme cold conditions in eastern North America [Wang et al., 2014b].
To further quantify the similarity between circulation during the winters of 1933–1934
and 2013–2014, we computed centered anomaly correlations (ACc) over North America (170°W–50°W, 20°N–70°N) between monthly 500 hPa height fields for these two periods. Of all months during the water year of 1933–1934, November 1933 and March 1934 were the strongest circulation analogues to this most recent winter.
Highest correlations with November 1933 are in November 2013 (ACc = +0.51),
December 2013 (ACc = +0.58), January 2014 (ACc = +0.74), and March 2014 (ACc = +0.57). For March 1934, correlations are similarly strong with December 2013 (ACc = +0.56), January 2014 (ACc = +0.68), and March 2014 (ACc = +0.64).
November 1933 also correlates strongly with circulation during October–December 1976 (ACc = +0.64 to +0.71), the beginning of one of the most severe Californian droughts in the historical record (1976–1977). While further analysis is beyond the scope of this paper, these correlations do suggest that wintertime ridging along the West Coast may play a recurring role in driving precipitation deficits during major West Coast droughts.
The 1933–1934 geopotential height and climate anomalies are not consistent with a
La Niña SST forcing signal; Wang et al. [2014b], however, linked the 2013–2014 winter circulation pattern to SST variability preceding an El Niño event in the western tropical Pacific (110°E–160°E, 10°S–20°N). Compared to the winter of 2013–2014, SST anomalies in this region were relatively weak during 1933–1934.
To more explicitly test the SST forcing hypothesis, however, we analyzed ensemble simulations of two atmospheric models forced by observed SSTs, simulations that will contain responses to precursor SST anomalies, including La Niña conditions and other global SST patterns. These models (the Community Climate Model Version 3 (CCM3)
and the NASA Goddard Earth Observing System model version 5 (GEOS-5)) have been used previously to investigate SST forcing of North American drought variability
[e.g., Seager et al., 200]